- Details
ANSTO has secured $80.2 million in new funding to expand the research capabilities of the Australian Synchrotron.
The funding boost was made by the New Zealand Synchrotron Group Limited (representing funding from the New Zealand Government and 10 New Zealand universities and research institutions), the Defence Science and Technology Group and 19 universities and medical research institutes across Australia.
The new funding will expand the number of beamlines at the Synchrotron from 10 to as many as 18, increasing research output at the facility and helping keep up with significant researcher demand for the state-of-the-art facility.
The first stage of the expansion will see the construction of the Micro-computed Tomography (MCT) beamline and the Medium Energy XAS (MEX) beamline:
• The MCT beamline will complement the Imaging and Medical Beamline (IMBL), by allowing 3D structures to be studied in close detail, which will enable advanced research in the fields of biological and health sciences.
• The MEX beamline will enable mapping of lighter elements such as sulphur, phosphorous, chlorine, calcium and potassium, with applications across sectors including aiding in the development of cancer treatment.
These beamlines will be closely followed by a Small Angle X-ray Scattering (BioSAXS) beamline. Supported by the New Zealand Synchrotron Group’s significant $25 million investment, the beamline will allow for detailed protein studies focussed on improving drug design and validation processes.
Minister for Industry, Innovation and Science, Senator the Hon Arthur Sinodinos, welcomed the funding for the beamline expansion, which will be supported by the Australian Government’s significant operational investment made via the National Innovation and Science Agenda (NISA).
The NISA provides $520 million in operational funding to the Australian Synchrotron, which includes operational funding for the new beamlines.
Minister Sinodinos said the scale of the contributions highlighted the extremely significant role the Synchrotron plays in Australia’s science and innovation ecosystem.
“The Australian Synchrotron is one of our most important pieces of landmark research infrastructure, which on a daily basis delivers practical benefits across a variety of vital areas,” Minister Sinodinos said.
“This is applied science at its best, with applications for medical researchers, the environment and industry.
“The Australian Government welcomes this new investment to expand capacity at the Synchrotron, which will be supported by our significant operational investment made via the National Innovation and Science Agenda (NISA).”
ANSTO CEO, Dr Adi Paterson, said this was the latest ANSTO collaboration that provides new opportunities for industry and researchers.
“ANSTO has been working to secure more than $100 million in capital funding to ensure the facility remains world-class and continues to meet the needs of researchers and industry,” Dr Paterson said.
“This is a great first step, and we look forward to continuing to work with industry and government to support the vitally important work of our scientists and researchers.”
Australian Synchrotron Director, Professor Andrew Peele said the expansion will alleviate demand issues and enable new research opportunities.
“This expansion will give Australian and New Zealand industry and our best and brightest scientific minds access to even more specialised tools and techniques needed for important research,” said Professor Peele.
“This will enable them to continue to compete on the world-stage and deliver real-life benefits to the community.”
ANSTO will continue to work with universities and other stakeholders to secure the remainder of the required funding.
Contributors to the project include:
Australian National University - Charles Sturt University - Curtin University – Deakin University - Macquarie University – Monash University - Queensland University of Technology - RMIT University - Swinburne University - University of Canberra - University of Melbourne - University of New South Wales - University of Queensland - University of the Sunshine Coast - University of Sydney - University of Tasmania - University of Western Australia - University of Wollongong – Walter and Eliza Hall Institute of Medical Research – ANSTO - Defence Science and Technology Group - New Zealand Synchrotron Group (including contributions from the New Zealand government and 10 universities and research institutions)
Media enquiries:
Minister Sinodinos: Nat Openshaw 0409 049 128
ANSTO / Australian Synchrotron: Phil McCall 0438 619 987
- Details
Queensland researchers have shown that single crystals, typically thought of as brittle and inelastic, are flexible enough to be bent repeatedly and even tied in a knot.
Researchers from Queensland University of Technology and The University of Queensland (UQ) determined and measured the structural mechanism behind the elasticity of the crystals down to the atomic level using the Australian Synchrotron.
Video: Copyright QUT
Their work, published in Nature Chemistry, opens the door for the use of flexible crystals in applications in industry and technology.
The research was led by ARC Future Fellows Associate Professor Jack Clegg in UQ’s School of Chemistry and Molecular Biosciences and Associate Professor John McMurtrie in QUT’s Science and Engineering Faculty.
Associate Professor McMurtrie said the results challenged conventional thinking about crystalline structures.
“Crystals are something we work with a lot – they’re typically grown in small blocks, are hard and brittle, and when struck or bent they crack or shatter,” he said.
“While it has previously been observed that some crystals could bend, this is the first study to examine the process in detail.
“We found that the crystals exhibit traditional characteristics of not only hard matter, but soft matter like nylon.”
The researchers grew bendable crystals about the width of a fishing line and up to five centimetres long from a common metal compound – copper (II) acetylacetonate.
They mapped changes in the atomic scale structure when the crystals were bent using X-ray measurements performed at the Australian Synchrotron.
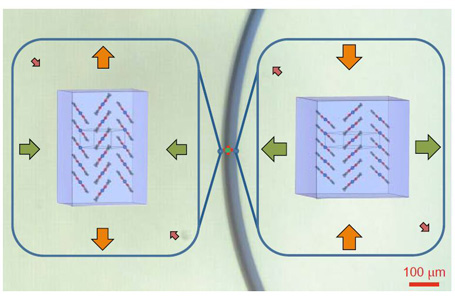
Dr Jason Price, a beamline scientist at the Australian Synchrotron, who helped design the experiments, explained that preliminary studies were undertaken on the MX1 beamline and followed by extensive measurements on the MX2 beamline.
“This experiment really shows the opportunity to look at variation within a single crystal. The microfocus capacity of the beam at MX2 is such that it can gather data from different parts of the crystal, so, when it is under strain from expansion or compression.”
Crystals from six other structurally related compounds, some containing copper and some other metals, were also tested and found to be flexible.
Associate Professor Clegg said the experiments showed that the crystals can be repeatedly bent and return quickly to their original shape with no signs of breaking or cracking when the force bending them is removed.
“Under strain the crystal molecules reversibly rotate and reorganise to allow the compression and expansion required for elasticity and still maintain the integrity of the crystal structure,” he said.
“The ability of crystals to bend flexibly had wide-ranging implications in industry and technology.
“Crystallinity is a property that underpins a variety of existing technologies, including semi-conductors and lasers which are used in almost every electronic device from DVD players to mobile phones and computers.
“But the hardness that makes them suitable for high-strength industrial components limits their use in other technologies. Flexible crystals like these could lead to new hybrid materials for numerous applications from components of planes and spacecraft to parts of motion or pressure sensors and electronic devices.”
Associate Professor McMurtrie said the method the researchers have developed to measure the changes during bending could also be used to explore flexibility in any other crystals.
“This is an exciting prospect given that there are millions of different types of crystals already known and many more yet to be discovered,” he said.
“Bending the crystal changes its optical and magnetic properties, and our next step is to explore these optical and magnetic responses with a view to identifying applications in new technologies.”
The research was funded by an ARC Discovery Grant and supported by the Australian Synchrotron. Research collaborators and co-authors of the study are: Anna Worthy, Professor Chen Yan, and Yanan Xu (QUT) and Dr Arnaud Grosjean, Dr Michael Pfrunder and Dr Grant Edwards (UQ).
http://dx.doi.org/10.1038/nchem.2848
For interview:
QUT Associate Professor John McMurtrie, j.mcmurtrie@qut.edu.au 07 3138 1220
UQ Associate Professor Jack Clegg, j.clegg@uq.edu.au 0408 642082
- Details
International researchers led by Assoc Prof Chris McNeill’s group at Monash University have used an X-ray scattering at the Australian Synchrotron to understand how microstructure contributes to the performance of an organic solar cell made with a semiconducting polymer and fullerene thin film.
The investigators evaluated the performance, microstructure and photophysics of dual stack bulk heterojunction (BH) solar cells made with a low bandgap polymer and fullerene thin film in a study published in Advanced Energy Materials.
The research relied on a range of techniques including X-ray scattering, photoluminescence spectroscopy, ultrafast transient absorption spectroscopy and transient photovoltage measurements, to gain a better understanding of how the choice of fullerene acceptor influences microstructure, photophysics and contribute to device performance.
A bulk heterojunction device is fabricated by coating a blend of two organic semiconductors between two electrodes. Thin films, which are usually less than 100 nanometres thick, offer production advantages for solar cells.
The close blending of materials is required for high performance because photo-generated excitons travel less than 10 nanometres before recombining, with photocurrent generation proceeding through via the dissociation of excitons a material interfaces. Efficient devices therefore require the blend to be optimally structured on the nanoscale.
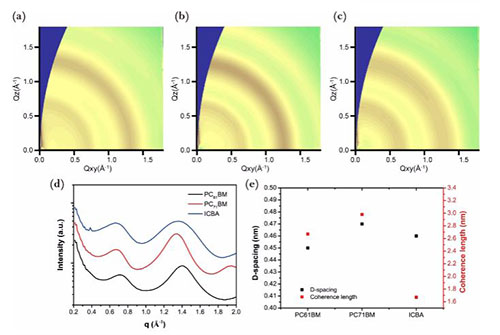
Advanced characterisation techniques are used to understand how microstructure, or morphology, contributes to power conversion efficiency.
Fullerenes act as electron acceptors in a device. Three novel compounds, PC71BM, PC61BM and ICBA were blended with a low band gap polymer, PBDTTT-EFT for the study.
The highest efficiency (9.4%) was found in the blend using the acceptor PC71BM, which also had the highest visible light absorption.
Prof Chris McNeill and collaborators used Synchrotron-based grazing incidence wide angle X-ray scattering (GIWAXS) to determine the molecular orientation of the polymer with respect to the substrate in the bulk of the thin film.
The GIWAXS measurements provided information about the orientation of polymer crystallites in the bulk, with these crystallites needing to be properly aligned in order for charges to travel through the material more easily.
Resonant soft X-ray scattering was also carried out by co-author Dr Lars Thomsen at the Advanced Light Source at Lawrence Berkley National Laboratory in the US to clarify the structure and purity of domains.
Thomsen, a member the soft x-ray spectroscopy beamline team at the Synchrotron, has undertaken previous collaborations with the McNeill Group on organic electronics.
R-SoXS indicated that one of the blends, PBDTTT-EFT:PC71BM exhibited the largest domain size and highest domain purity, which was thought to facilitate charge separation and transport.
The scattering profiles also provided an indication of the roughness of the interfaces between the domains in the three compounds.
The R-SoXS and other methods suggested that the lower power conversion efficiency of the ICBA compound might be explained by rougher domain interfaces, lower crystallinity and smaller domain size.
Contributors include Dr Wenchao Huang and colleagues at Monash University, Dr Eliot Gann (now at Brookhaven National Lab), researchers from the University of California Los Angeles, the Indian Institute of Technology Bombay and Victoria University of Wellington.