- Details
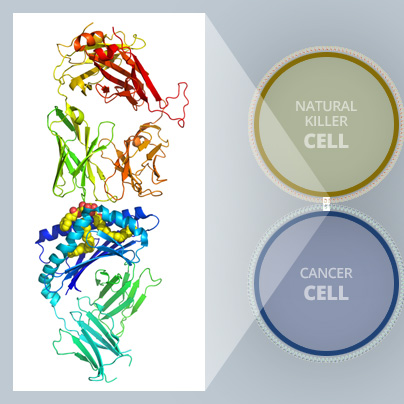
A subset of natural killer cells known as invariant natural killer T (iNKT) cells are powerful weapons our body’s immune systems count on to fight infection and combat diseases like cancer, multiple sclerosis, and lupus. Finding ways to spark these potent cells into action could lead to more effective cancer treatments and vaccines.
While several chemical compounds have shown promise stimulating iiNKT cells in animal models, their ability to activate human iNKT cells has been limited.
Now, an international team of top immunologists, structural biologists, and chemists published in Cell Chemical Biology the creation of a new compound that appears to have the properties researchers have been looking for. The research was co-led by Monash Biomedicine Discovery Institute’s (BDI) Dr Jérôme Le Nours, University of Connecticut’s Professor Amy Howell and Albert Einstein College of Medicine’s Dr Steve Porcelli. Dr Le Nours used the Micro Crystallography beamline (MX2) at the Australian Synchrotron as part of the study.
The compound – a modified version of an earlier synthesized ligand – is highly effective in activating human iNKT cells. It is also selective – encouraging iNKT cells to release a specific set of proteins known as Th1 cytokines, which stimulate anti-tumour immunity.
One of the limitations of earlier compounds was their tendency to cause iNKT cells to release a rush of different cytokines. Some of the cytokines turned the body’s immune response on, while others turned it off. The conflicting cytokine activity hampered the compound’s’ effectiveness.
The new compound – called AH10-7 – is uniquely structured so that does not happen.
“One of the goals in this field has been to identify compounds that elicit a more selective response from iNKT cells, and we were able to incorporate features in AH10-7 that did that,” Professor Howell said.
The robust study, years in the making, also applied advanced structural and 3D computer modelling analysis to identify the underlying basis for the new compound’s success.
These highly detailed insights into what is happening at the molecular level open up new paths for research and could lead to the development of even more effective compounds (see illustration above).
Using the MX2 beamline as one of the experimental methods in the study, co-leading author Dr Jérôme Le Nours, a structural biologist and ARC Future fellow within the Biomedicine Discovery Institute at Monash University, obtained atomic structures of the key proteins revealing detailed molecular interactions. The molecular analysis helped validate and explain experimental results.
“By exposing a crystalized form of the molecular complex to a high-intensity X-ray beam at the Australian Synchrotron, we were able to obtain a detailed 3-D image of the molecular interplay between the invariant natural killer T cell receptor and AH10-7,” Dr Le Nours said.
“This enabled us to identify the structural factors responsible for AH10-7’s potency in activating iNKT cells. This valuable insight could lead to the development of even more effective anti-metastatic ligands,” he said.
Principal scientist of the macromolecular crystallography beamlines, Dr Tom Caradoc-Davies, who provides technical support on the instrument, said the research represented a significant accomplishment based on a painstaking multi-disciplinary contribution from around the globe and was a promising development.
Efforts to harness the therapeutic potential of human iNKT cells began 20 years ago with the discovery that natural and synthetic forms of glycolipid ligands known as alpha-galactosylceramides, or α-GalCers for short, were potent activators of iNKT cells.
Scientists immediately recognized their possible value in fighting cancer and other diseases. These α-GalCer ligands serve as tiny dock masters in our immune system, helping antigen-presenting cells attract and bind with iNKT cells so they can be activated to kill cancerous cells or fight off pathogens and other foreign invaders.
The first promising version of a synthesized α-GalCer was a compound known as KRN7000. While KRN7000 powerfully stimulated iNKT cells, it triggered the release of a flood of many types of cytokines, limiting its potential for clinical applications.
Since then, researchers have been searching for new variations of KRN7000 that maintain their effectiveness in activating human iNKT cells while also favouring release of the powerful tumour fighting Th1 cytokines.
In the current study, the research team made two significant structural modifications to the α-GalCer ligand in an attempt to make it more effective.
Both changes, working in tandem, strengthened the effectiveness of the entire molecular complex in terms of activating human iNKT cells, Professor Howell says.
This article is based on a media release originally prepared by the University of Connecticut.
Read the full paper: Cell Chemical Biology titled Dual Modifications of α-Galatosylceramide Synergize to Promote Activation of Human Invariant Natural Killer T Cells and Stimulate Anti-tumor Immunity.
The research was supported in part by grants from the National Institutes of Health in the US.
- Details
The Australian Synchrotron is proud to be growing Australia’s capacity for innovative drug development, facilitating the advance of world-class disease and drug research through to local drug trials. Recent success in clinical trials of Venetoclax, the chronic lymphocytic leukaemia (CLL) drug developed by researchers from the Walter and Eliza Hall Institute and two international pharmaceutical companies is driving a major shift in the treatment of a range of blood cancers, according to a media information from the Peter MacCallum Cancer Centre. A clinical trial testing the drug for CLL is being undertaken at the Peter MacCallum Cancer Centre and the Royal Melbourne Hospital. The drug is also being tested for a type of lymphoma in the US. Micro-crystallography on the MX2 beamline was used for structural biology studies of the drug during development. Read more on Peter MacCallum Cancer Centre website report. Read about how the Australian Synchrotron contributed to the research that led to the development of the drug in this report.
- Details
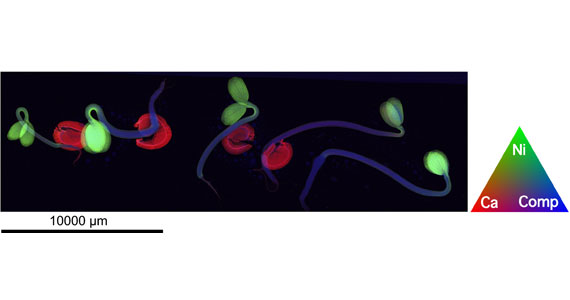
The complementary power of combining multiple X-ray techniques to understand the unusual properties of hyperaccumulator plants has been highlighted in a new cover article just published in New Phytologist.
X-ray fluorescence microscopy (XFM) at the Australian Synchrotron has been used by a consortium of international researchers led by Dr Antony van der Ent of the Centre for Mined Land Rehabilitation at The University of Queensland, in association with A/Prof Peter Kopittke of the School of Agriculture and Food Science also at The University of Queensland.
The XFM technique generates elemental maps showing where elements of interest are found within plant tissue, seedlings or individual cells.
Visually striking images (obtained at the XFM beamline) show various hyperaccumulator plants, on the cover of the April issue of New Phytologist. In the images each element is depicted in a different colour, making up a red-green-blue (RGB) image.
“Hyperaccumulator plants have the unusual ability to accumulate extreme concentrations of metals and metalloids in their living tissues,” said van der Ent.
“Hyperaccumulators are of scientific interest because whilst metals are normally toxic to plants even at low concentrations, these plants are able to accumulate large concentrations without any toxic effects,” he added
“The question is ‘how are they able to do that and what is the mechanism which enables them to tolerate such high metal concentrations?’ Is there something we can learn from in terms of trying to understand metal toxicity in normal plants— particularly in those we use in agriculture?”
“Studies of the hyperaccumulators are also undertaken for applications in phytomining (a novel approach to involves cultivating hyperaccumulator plants as ‘metal crops’ to produce valuable metals),” said van der Ent.
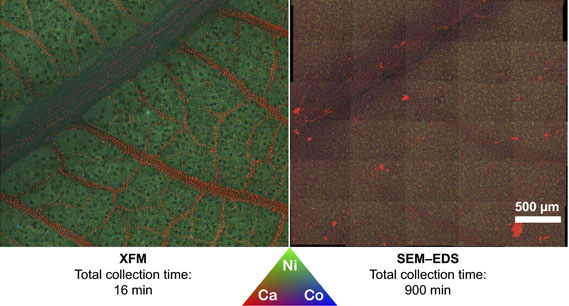
XFM beamline scientist Dr Martin de Jonge, a co-author on the paper with Dr David Paterson, said the technique was used to study how metals are taken up in roots and distributed throughout the plant under ‘lifelike’ conditions.
Among the different X-ray techniques that were evaluated in the paper, each has its own advantages. Kopittke said he was a strong proponent of XFM for this very reason.
“Because a vacuum is not required, you can undertake the experiment in ambient conditions. Plants are more than 90 per cent water and if you have to dehydrate them to put them in a vacuum as you do for other X-ray techniques, you invariably move the elements you are analysing. With XFM we can analyse a live plant in its natural state, and this is highly advantageous. You cannot do this with any other technique, “said Kopittke.
The authors note that as XFM can probe to a depth from several hundred micrometres to the millimetre scale, it is ideal for addressing plant science. “Its high sensitivity and capacity to be used on large samples make it very versatile,” said de Jonge.
Unique to the XFM beamline is the use of a revolutionary X-ray detector Maia which is an extremely fast detector system. Maia makes it possible to obtain elemental maps that are many millions of pixels (megapixels) in size in hours. Because of this, there was no visible evidence of radiation-induced damage to live plants.
XFM can simultaneously map hyperaccumulated metals (such as nickel), important nutritional elements (such as potassium) and trace elements that are typically present at far lower concentrations (such as copper).
“You can illuminate with a single energy and you get the fluorescence from everything, known as the spectral axis,” said de Jonge. “But you can also modify the incident energy and look at changes in the spectral axis as a result of that.This helps you probe the electronic structure of atoms.“
The other techniques that were comprehensively reviewed in the paper included proton induced X-ray emission (PIXE). This technique can be undertaken on frozen samples; thereby making it possible to investigate cross-sections of plant tissue to elucidate the internal cellular-level distribution of elements. PIXE is also ideally suited to measure very light elements, such as aluminium.
“The complementarity of X-ray elemental mapping techniques provides a way to answering questions at every level about how plants take up metals and maintain their physiological functions” said Kopittke.
Collaborating institutions included The University of Queensland, University of Adelaide, Université de Lorraine (France), iTHEMBA Labs (South Africa), University of Science and Technology (Poland), Jagiellonian University (Poland), and CSIRO.
https://doi.org/10.1111/nph.14810