- Details
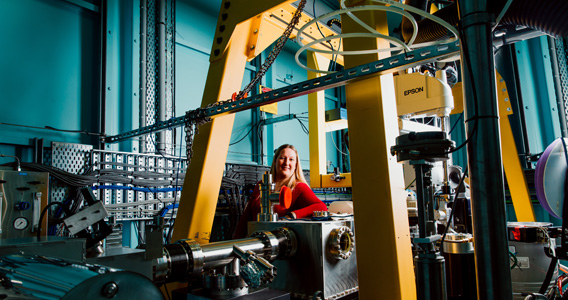
While treatments for HIV mean that the disease is no longer largely fatal, the world still lacks a true therapy that can eradicate the virus across a globally – and genetically different – population.
Monash researchers, together with colleagues from the Pasteur Institute in Paris, have discovered a unique set of “super” receptors on immune cells capable of killing HIV across genetically diverse populations, making them a potential candidate for immunotherapy treatments. The work was recently published in Science Immunology.
Associate Professor Stephanie Gras and her team from the Monash University Biomedicine Discovery Institute and ARC Centre of Advanced Molecular Imaging, and her colleagues from the Pasteur Institute in Paris, studied fifteen unique individuals who all had been infected with HIV (ANRS CO21 CODEX cohort), but have immune system that protect them from AIDs progression. These rare individuals, called HIV controllers, could hold clues to the cure for the disease.
Upon HIV infection, CD4 T cells, which are an important part of our protective immune system, can be depleted and drop dramatically in numbers, leading to a weak immune system with the progression of the disease to AIDs. These CD4 T cells can remain low even when the disease is kept in check with anti-retroviral therapy (ART), which is currently provided to more than half of people living with HIV globally. ART lowers the risk of mortality but does not eradicate the virus.
Associate Professor Gras and her colleagues found that HIV controllers are able to retain CD4 T cells of a higher quality, able to detect and react to minute amounts of virus, therefore representing a great opportunity to study their potential role in HIV infection.
We discovered that those CD4 T cells, usually viewed as helper cells for the killer CD8 T cells that destroy infected cells, could be turned into killer cells themselves in HIV controllers. These killer CD4+ T cells could recognize very low amounts of HIV thanks to the expression of “super” T cell receptors on their surface. Importantly when they studied these receptors – they found identical receptors across multiple HIV controllers. “The likelihood of finding the exact same T cell receptor in different individuals is extremely low, like winning the lottery, and is likely playing a role in the control of HIV” Carine Farenc, a lead author of the study said.
T cell receptors recognize virus or bacteria fragments bound to specialized molecule called HLA (Human Leukocyte Antigen). HLA molecules are like fingerprints: every person has a specific combination of HLA molecules, which help the immune system recognize foreign invaders like bacteria or viruses.
Monash University researchers used the Australian Synchrotron, effectively a giant microscope the size of a football field, to study the binding of this super T cell receptor in complex with the HIV antigen.
“I looked at the T-cell receptor or TCR and how the TCR is able to recognise the HIV peptide. When we get infected with the HIV virus, the virus will break down into small pieces, peptides, and the peptide will be loaded on to HLA molecule. The HLA molecule is able to present small antigen to the T cell receptor. So using MX1 and MX2, we have been able to look at the interaction between the TCR and the HIV peptide presented by the HLA molecule," said Associate Professor Gras.
“And there is something quite remarkable about our TCR, in that this TCR is able to recognise the same HIV peptide presented by different HLA molecules. HLA molecule is like a fingerprint, we all have different HLA molecules. Each is quite unique. Usually one TCR recognises the HLA molecule but this TCR that we found is able to recognise a lot of different molecules. And using the synchrotron we were able to understand how this was done by the TCR. “
The Gras team and her colleagues found that these killer CD4 T cells can bind with HLA molecules shared by a quarter of world population, a figure that is likely to increase as studies progress, according to Associate Professor Gras.
http://immunology.sciencemag.org/content/3/24/eaat0687
Statistics (from WHO):
In 2016:
• there were 36.7 million people living with HIV
• 1 million died from the disease
• 19.5 million people living with HIV were receiving antiretroviral treatment (ART)
Content provided by Monash University
- Details
Walter and Eliza Hall Institute researchers have visualised for the first time how the protein SOCS1 ‘switches off’ cell signalling to dampen immune responses and block cancer growth.
The atomic-level structure of SOCS1 binding to its partner protein JAK could guide the development of drugs that alter disease-causing cell signalling pathways, and may have applications for treating some blood cancers, including leukaemias.
Crystallisation and structure determination of JAK1/SOCS1 was undertaken on the Australian Synchrotron's MX2 beamline.
The research, led by Dr Nick Liau, Dr Nadia Kershaw, Associate Professor Jeff Babon and Professor Nick Nicola, was published in the journal Nature Communications.
At a glance
- The SOCS1 protein binds to JAK proteins to ‘switch off’ cell signalling, which dampens processes including immune responses and cancer growth.
- Our researchers have used structural biology to visualise how SOCS1 binds to JAK proteins in never-before seen detail.
- The detailed structure may guide the development of new drugs that modify JAK activity, amplifying or dampening cell responses, with potential applications in cancer therapies.
Switching off signalling
Dr Liau said the structure of the protein pair revealed for the first time how SOCS1 binds to JAK proteins to disable signalling.
“Using the Australian Synchrotron and the CSIRO Collaborative Crystallisation Centre, we produced an incredibly detailed view of how SOCS1 interacts with the JAK1 protein,” Dr Liau said. “With this image, we were able to explain for the first time why JAK proteins cannot signal when bound to SOCS1. This information could help to underpin the development of new medicine targeting this important cell signalling pathway.”
Above: The structure of SOCS1 (red) bound to a JAK protein (beige) could inform the development of new drugs to treat cancer. Video courtesy of Walter and Eliza Hall Institute
A blueprint for new medicines
Dr Kershaw said both SOCS1 and JAK proteins had been implicated in driving diseases including cancer and inflammatory conditions.
“In particular, overactive JAK signalling is linked to the development of cancer-like conditions called myeloproliferative neoplasms (MPNs) – which include polycythemia vera, essential thrombocythemia and primary myelofibrosis – as well as certain acute childhood leukaemias.
“Medicines that inhibit JAK signalling are in use for treating MPNs, but they are only able to manage the disease, not cure it. New medicines for these conditions are needed, and we envisage that a drug designed to mimic the SOCS1 protein to switch off JAK proteins might be a more effective treatment,” Dr Kershaw said.
MichaelAs well as guiding the development of drugs mimicking SOCS1, the team’s research may also underpin the development of a second new class of drugs that inhibit SOCS1, Associate Professor Jeff Babon said. “SOCS1 binding JAK proteins normally applies a ‘brake’ to immune responses – which in a healthy person is a good thing,” he said.
“However, in certain conditions, releasing this brake could be the key to enhanced immune responses. This approach to boosting the immune response could be the key to improving immunotherapies for treating cancer. If we could design a drug that inhibits SOCS1, this might boost anti-cancer immune responses, potentially improving anti-cancer immunotherapies.”
Dr Liau is a former PhD student at the Institute, enrolled through the University of Melbourne’s Department of Medical Biology. The research was supported by Cancer Council Victoria, National Health and Medical Research Council and the Victorian Government.
Content provided by Walter and Eliza Hall Institute
- Details
Australian researchers have made a surprise discovery that could rewrite our understanding of the role programmed cell death plays in embryonic development and congenital birth defects.
The team showed that, while programmed cell death – or apoptosis – is essential for healthy development overall, many organs and tissues do not require apoptosis to develop normally.
The study, published today in the high-ranking journal Cell, also suggested that abnormalities in cell death processes are likely to contribute to some common birth defects in humans, such as spina bifida, heart vessel defects and cleft palate.
Walter and Eliza Hall Institute researchers Dr Francine Ke, Dr Hannah Vanyai, Dr Angus Cowan, Associate Professor Anne Voss and Professor Andreas Strasser led the research.
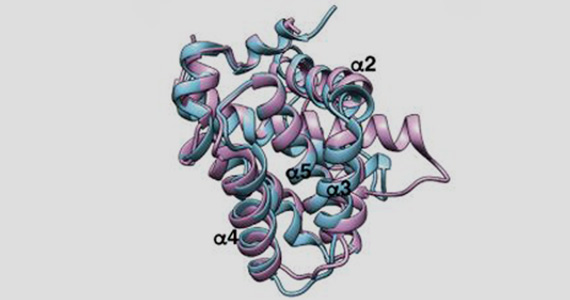
Experiments to determine the crystal structure of a key 'pro-death' protein, BOK, were carried out using the MX beamline at the Australian Synchrotron.
Cell death link to birth defects
Programmed cell death, also known as apoptosis, is a normal process that rids the body of sick, damaged or unwanted cells in a controlled way, limiting side effects and damage to the body.
Apoptosis was first described as having a role in embryonic development in the 1940s. Over the past 70 years, numerous studies have implicated apoptosis as playing a crucial role in most stages and tissues during development.
However, in this new study, it became clear that apoptosis was not as critical during development as previously thought, Dr Ke said.
“Rather, apoptosis was essential at specific places and times during development, but unnecessary in others. We identified the tissues and organs that critically require apoptosis to develop normally, and made the surprise discovery that many do not require it at all,” Dr Ke said.
The finding provides clear clues about a link between abnormalities in programmed cell death and some common congenital birth defects, including spina bifida, heart vessel defects and cleft palate.
“Our research showed that when cell death is not functioning properly, it commonly leads to defects in neural tube development, for example spina bifida, heart vessel defects and facial abnormalities, such as cleft palate,” Dr Ke said.
Surprise discovery
Associate Professor Voss said she thought the biggest surprise came from the discovery of the tissues that did not require apoptosis at all for normal development.
Dr Cowan said the study confirmed that BOK acted as a pro-death protein. “In this paper we have solved the structure of BOK using the Australian Synchrotron, and once and for all confirmed that BOK is a pro-death protein that plays an important role in apoptosis,” he said.
“For some time, it has been a widely-held belief that programmed cell death is necessary for the shaping of certain tissues and structures during development. But, to our surprise, many tissues in which programmed cell death was – for very good reasons – considered absolutely essential, it is not required at all," Associate Professor Voss said.
“For example, apoptosis was thought to play a particularly important role in ‘hollowing out’ of ducts in internal organs during development.
"However we have shown that – in the absence of apoptosis – most tissues and organs develop normally. I think it may surprise researchers to learn just how precise and limited the effects of apoptosis are in embryonic development."
The research was supported by the Australian National Health and Medical Research Council, Cancer Council Victoria, Lady Tata Memorial Trust, Leukaemia Foundation of Australia, Leukemia & Lymphoma Society (US) and the Victorian Government.